Genomes by design
Arc’s Hsu Lab uncovers the first natural RNA-guided recombinase that can programmably insert, excise, or invert any two DNA sequences of interest
Over the last quarter-century, RNA-guided systems have transformed our ability to program biology. Relative to the complexity of designing an entirely new protein or small molecule binder from scratch, an RNA-guided molecular machine can be redirected to a completely new target by simply changing a short stretch of RNA nucleotides. This approach was first widely adopted following the discovery of RNA interference in the late 1990s, and then accelerated by the gene editing revolution instigated by CRISPR in the early 2010s. However, both of these RNA-guided systems naturally cut, damage or destroy their DNA or RNA targets to inactivate them.
In a quest over the last two and a half years, scientists at the Arc Institute have now discovered a compact and entirely new type of programmable molecular system that evolved to directly insert new DNA sequences into the genome using bridge RNAs – a new class of guide RNAs.
The study, published today in Nature, reports their discovery of the first DNA recombinase that uses a non-coding RNA for sequence-specific selection of target and donor DNA molecules. This bridge RNA is programmable, allowing the user to specify any desired genomic target sequence and any donor DNA molecule to be inserted.
"The bridge RNA system is a fundamentally new mechanism for genome design," said Dr. Patrick Hsu, senior author of the study and an Arc Institute Core Investigator and University of California, Berkeley Assistant Professor of Bioengineering. "Bridge recombination can universally modify genetic material through sequence-specific insertion, excision, inversion, and more, enabling a word processor for the living genome beyond CRISPR."
Arc senior scientist Dr. Matthew Durrant and UC Berkeley bioengineering graduate student Nicholas Perry were the lead authors of the discovery. The research was developed in collaboration with the labs of Dr. Silvana Konermann, Arc Institute Core Investigator and Stanford University Assistant Professor of Biochemistry, and Dr. Hiroshi Nishimasu, Professor of Structural Biology at the University of Tokyo.
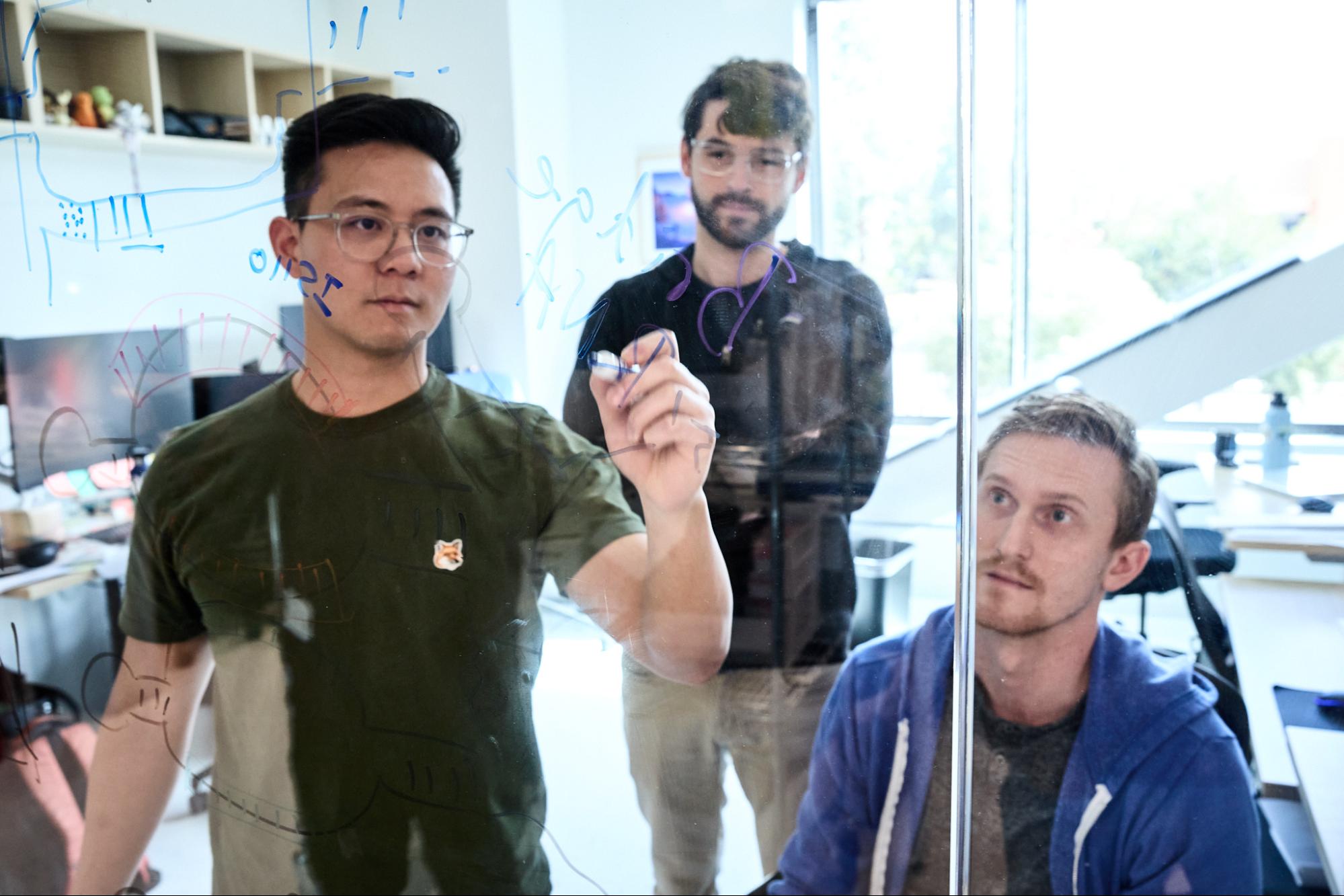
Programmable RNA
The bridge recombination system hails from insertion sequence 110 (IS110) elements, one of countless types of transposable elements—or “jumping genes”—that cut and paste themselves to move within and between microbial genomes. Transposable elements are found across all life forms and have evolved into professional DNA manipulation machines in order to survive. The IS110 elements are very minimal, consisting only of a gene encoding the recombinase enzyme, plus flanking DNA segments that have, until now, remained a mystery.
The Hsu lab found that when IS110 excises itself from a genome, the non-coding DNA ends are joined together to produce an RNA molecule – the bridge RNA – that folds into two loops. One loop binds to the IS110 element itself, while the other loop binds to the target DNA where the element will be inserted. The bridge RNA is the first example of a bispecific guide molecule, specifying the sequence of both target and donor DNA through base-pairing interactions.
Each loop of the bridge RNA is independently programmable, allowing researchers to mix and match any target and donor DNA sequences of interest. This means the system can go far beyond its natural role that inserts the IS110 element itself, instead enabling insertion of any desirable genetic cargo—like a functional copy of a faulty, disease-causing gene—into any genomic location. In this work, the team demonstrated over 60% insertion efficiency of a desired gene in E. coli with over 94% specificity for the correct genomic location.
“These programmable bridge RNAs distinguish IS110 from other known recombinases, which lack an RNA component and cannot be programmed,” said Perry. “It’s as if the bridge RNA were a universal power adapter that makes IS110 compatible with any outlet.”
The study is complemented by their collaboration with the lab of Dr. Hiroshi Nishimasu at the University of Tokyo, also published today in Nature. The Nishimasu lab used cryo-electron microscopy to determine the molecular structures of the recombinase-bridge RNA complex bound to target and donor DNA, sequentially progressing through the key steps of the recombination process.
With further exploration and development, the bridge mechanism promises to usher in a third generation of RNA-guided systems, expanding beyond the DNA and RNA cutting mechanisms of CRISPR and RNA interference (RNAi) to offer a unified mechanism for programmable DNA rearrangements. Critical for the further development of the bridge recombination system for mammalian genome design, the bridge recombinase joins both DNA strands without releasing cut DNA ends—sidestepping a key limitation of current state-of-the-art genome editing technologies.
“In addition to the discovery of a new molecular mechanism of RNA function, the bridge recombination mechanism has the potential to solve some of the most fundamental challenges facing other methods of genome editing,” said research co-lead Durrant. “The ability to programmably rearrange any two DNA molecules opens the door to breakthroughs in genome design.”
Building the future of genome design
Bridge recombination provides a springboard for further exploration of its potential applications in genome design. Future areas of research include adapting the system for use in human cells, boosting its precision and efficiency, and exploring additional IS110 element functionalities—like how it excises itself from the genome after its editing work is complete —that could have ripple effects for other genome design tools.
These refinements to the bridge RNA system could supercharge Arc’s multidisciplinary quest to understand and manipulate how genes interact within complex networks. In the realm of genome design, computational scientists could deploy bridge RNAs to validate synthetic genomic research into the language, syntax, and grammar of genomes. Researchers could also use it as a functional genomics tool to make precise gene insertions at specific sites across the genome beyond single-gene disruption, opening new pathways for understanding how genes influence health and disease.
The Arc discovery is an early example of scientific output from the institute’s collaborative model, which blends expert scientific staff with graduate students from Arc’s partner universities–UC Berkeley, Stanford University, and UCSF–in an interdisciplinary research environment across computational and experimental science. “We are excited to explore new applications that stem from our team’s interdisciplinary research across computation, genetics, biochemistry, bioengineering, and structural biology—the deep synergistic work that Arc was specifically designed to accelerate," said Hsu.
Read the papers in Nature here and here. A short video of the discovery, developed in collaboration with Visual Science (X, LinkedIn, YouTube, Instagram), is available here.